chap 4-5
DownloadTélécharger
Actions
Vote :
ScreenshotAperçu
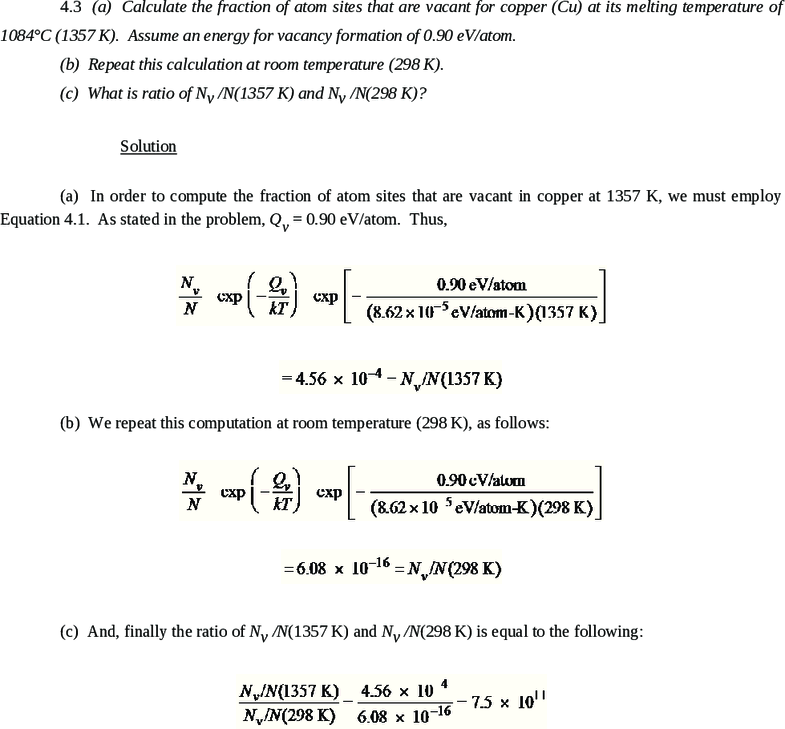
Informations
Catégorie :Category: mViewer GX Creator Lua TI-Nspire
Auteur Author: didierisaza
Type : Classeur 3.6
Page(s) : 20
Taille Size: 977.34 Ko KB
Mis en ligne Uploaded: 30/04/2015 - 09:06:56
Uploadeur Uploader: carl cox (Profil)
Téléchargements Downloads: 235
Visibilité Visibility: Archive publique
Shortlink : https://tipla.net/a205959
Type : Classeur 3.6
Page(s) : 20
Taille Size: 977.34 Ko KB
Mis en ligne Uploaded: 30/04/2015 - 09:06:56
Uploadeur Uploader: carl cox (Profil)
Téléchargements Downloads: 235
Visibilité Visibility: Archive publique
Shortlink : https://tipla.net/a205959
Description
4.3 (a) Calculate the fraction of atom sites that are vacant for copper (Cu) at its melting temperature of
1084°C (1357 K). Assume an energy for vacancy formation of 0.90 eV/atom.
(b) Repeat this calculation at room temperature (298 K).
(c) What is ratio of Nv /N(1357 K) and Nv /N(298 K)?
Solution
(a) In order to compute the fraction of atom sites that are vacant in copper at 1357 K, we must employ
Equation 4.1. As stated in the problem, Qv = 0.90 eV/atom. Thus,
(b) We repeat this computation at room temperature (298 K), as follows:
(c) And, finally the ratio of Nv /N(1357 K) and Nv /N(298 K) is equal to the following:
4.13 What is the composition, in atom percent, of an alloy that consists of 92.5 wt% Ag and 7.5 wt% Cu?
Solution
In order to compute composition, in atom percent, of a 92.5 wt% Ag-7.5 wt% Cu alloy, we employ
Equation 4.6 given the atomic weights of silver and copper (found on the inside of the book's cover):
AAg = 107.87 g/mol
ACu = 63.55 g/mol
These compositions in atom percent are determined as follows:
= 87.9 at%
= 12.1 at%
4.17 What is the composition, in atom percent, of an alloy that contains 33 g of copper and 47 g of zinc?
Solution
The concentration of an element in an alloy, in atom percent, may be computed using Equation 4.5a.
However, it first becomes necessary to compute the number of moles of both Cu and Zn, using Equation 4.4.
Atomic weights of copper and zinc (found on the inside of the book's cover) are as follows:
ACu = 63.55 g/mol
AZn = 65.41 g/mol
Thus, the number of moles of Cu is just
Likewise, for Zn
Now, use of Equation 4.5a yields
Also,
4.20 Calculate the number of atoms per cubic meter in Pb.
Solution
This problem calls for a determination of the number of atoms per cubic meter for lead. In order to solve
this problem, one must employ Equation 4.2,
The density of Pb (from the table inside of the front cover) is 11.35 g/cm3, while its atomic weight is 207.2 g/mol.
Thus,
4.21 Calculate the number of atoms per cubic meter in Cr.
Solution
This problem calls for a determination of the number of atoms per cubic meter for chromium. In order to
solve this problem, one must employ Equation 4.2,
The density of Cr (from the table inside of the front cover) is 7.19 g/cm3, while its atomic weight is 52.00 g/mol.
Thus,
4.25 Calculate the unit cell edge length for an 80 wt% Ag20 wt% Pd alloy. All of the palladium is in
solid solution, the crystal structure for this alloy is FCC, and the room-temperature density of Pd is 12.02 g/cm3.
Solution
In order to solve this problem it is necessary to employ Equation 3.8; in this expression density and atomic
weight will be averages for the alloy—that is
Inasmuch as the unit cell is cubic, then VC = a3, then
And solving this equation for the unit cell edge length, leads to
Expressions for Aave and Uaveare found in Equations 4.11a and 4.10a, respectively, which, when
incorporated into the above expression yields
Since the crystal structure is FCC, the value of n in the above expression is 4 atoms per unit cell. The
atomic weights for Ag and Pd are 107.87 and 106.4 g/mol, respectively (Figure 2.8), whereas the densities for the
Ag and Pd are 10.49 g/cm3 (inside front cover) and 12.02 g/cm3. Substitution of these, as well as the concentration
values stipulated in the problem statement, into the above equation gives
4.26 Some hypothetical alloy is composed of 25 wt% of metal A and 75 wt% of metal B. If the densities of
metals A and B are 6.17 and 8.00 g/cm3, respectively, and their respective atomic weights are 171.3 and 162.0
g/mol, determine whether the crystal structure for this alloy is simple cubic, face-centered cubic, or body-centered
cubic. Assume a unit cell edge length of 0.332 nm.
Solution
In order to solve this problem it is necessary to employ Equation 3.8; in this expression density and atomic
weight will be averages for the alloy—that is
Inasmuch as for each of the possible crystal structures, the unit cell is cubic, then VC = a3, or
Now, in order to determine the crystal structure it is necessary to solve for n, the number of atoms per unit
cell. For n =1, the crystal structure is simple cubic, whereas for n values of 2 and 4, the crystal structure will be
either BCC or FCC, respectively. When we solve the above expression for n the result is as follows:
Expressions for Aave and Uave are found in Equations 4.11a and 4.10a, respectively, which, when incorporated into
the above expression yields
Substitution of the concentration values (i.e., CA = 25 wt% and CB = 75 wt%) as well as values for the other
parameters given in the problem statement, into the above equation gives
= 1.00 atom/unit cell
Therefore, on the basis of this value, the crystal structure is simple cubic.
4.37 Iron and vanadium both have the BCC crystal structure, and V forms a substitutional solid solution
for concentrations up to approximately 20 wt% V at room temperature. Compute the unit cell edge length for a 90
wt% Fe–10 wt% V alloy.
Solution
This problems asks that we compute the unit cell edge length for a 90 wt% Fe-10 wt% V alloy. First of all,
the atomic radii for Fe and V (using the table inside the front cover) are 0.124 and 0.132 nm, respectively. Also,
using Equation 3.8 it is possible to compute the unit cell volume, and inasmuch as the unit cell is cubic, the unit cell
edge length is just the cube root of the volume. However, it is first necessary to calculate the density and average
atomic weight of this alloy using Equations 4.10a and 4.11a. Inasmuch as the densities of iron and vanadium are
7.87g/cm3 and 6.10 g/cm3, respectively, (as taken from inside the front cover), the average density is just
= 7.65 g/cm3
And for the average atomic weight
= 55.32 g/mol
Now, VC is determined from Equation 3.8 as (taking the number of atoms per unit cell, n = 2, since the crystal
structure of the alloy is BCC)
= 2.40 u 1023 cm3/unit cell
And, finally, because the unit cell is of cubic symmetry
= 2.89 u 108 cm = 0.289 nm
4.42 (a) For a given material, would you expect the surface energy to be greater than, the same as, or less
than the grain boundary energy? Why?
(b) The grain boundary energy of a small-angle grain boundary is less than for a high-angle one. Why is
this so?
Answer
(a) The surface energy will be greater than the grain boundary energy. For grain boundaries, some atoms
on one side of a boundary will bond to atoms on the other side; such is not the case for surface atoms. Therefore,
there will be fewer unsatisfied bonds along a grain boundary.
(b) The small-angle grain boundary energy is lower than for a high-angle one because more atoms bond
across the boundary for the small-angle, and, thus, there are fewer unsatisfied bonds.
Diffusion Mechanisms
5.3 (a) Compare interstitial and vacancy atomic mechanisms for diffusion.
(b) Cite two reasons why interstitial diffusion is normally more rapid than vacancy diffusion.
Answer
(a) With vacancy diffusion, atomic motion is from one lattice site to an adjacent vacancy. Self-diffusion
and the diffusion of substitutional impurities proceed via this mechanism. On the other hand, atomic motion is from
interstitial site to adjacent interstitial site for the interstitial diffusion mechanism.
(b) Interstitial diffusion is normally more rapid than vacancy diffusion because: (1) interstitial atoms,
being smaller, are more mobile; and (2) the probability of an empty adjacent interstitial site is greater than for a
vacancy adjacent to a host (or substitutional impurity) atom.
5.9 A sheet of steel 2.5-mm thick has nitrogen atmospheres on both sides at 900°C and is permitted to
achieve a steady-state diffusion condition. The diffusion coefficient for nitrogen in steel at this temperature is 1.85
× 10–10 m2/s, and the diffusion flux is found to be 1.0 × 10–7 kg/m2 . s. Also, it is known that the concentration of
nitrogen in the steel at the high-pressure surface is 2 kg/m3. How far into the sheet from this high-pressure side will
the concentration be 0.5 kg/m3? Assume a linear concentration profile.
Solution
This problem is solved by using Equation 5.2 in the form
If we take CA to be the point at which the concentration of nitrogen is 2 kg/m3, then it becomes necessary to solve
the above equation for xB, as
Assume xA is zero at the surface, in which case
5.14 An FCC iron–carbon alloy initially containing 0.55 wt% C is exposed to an oxygen-rich and virtually
carbon-free atmosphere at 1325 K (1052°C). Under these circumstances the carbon diffuses from the alloy and
reacts at the surface with the oxygen in the atmosphere—that is, the carbon concentration at the surface position is
maintained essentially at 0 wt% C. (This process of carbon depletion is termed decarburization.) At what position
will the carbon concentration be 0.25 wt% after a 10-h treatment? The value of D at 1325 K is 3.3 × 10–11 m2/s.
Solution
This problem asks that we determine the position at which the carbon concentration is 0.25 wt% after a 10-
h heat treatment at 1325 K when C0 = 0.55 wt% C. From Equation 5.5
Thus,
Using data in Table 5.1 and linear interpolation
z erf (z)
0.40 0.4284
z 0.4545
0.45 0.4755
which leads to,
z = 0.4277
This means that
And, finally
= 9.32 u 104 m = 0.932 mm
Note: This problem may also be solved using the “Diffusion” module in the VMSE software. Open the “Diffusion”
module, click on the “Diffusion Design” submodule, and then do the following:
1. Enter the given data in left-hand window that appears. In the window below the label “D Value” enter
the value of the diffusion coefficient—viz. “3.3e-11”.
2. In the window just below the label “Initial, C0” enter the initial concentration—viz. “0.55”.
3. In the window the lies below “Surface, Cs” enter the surface concentration—viz. “0”.
4. Then in the “Diffusion Time t” window enter the time in seconds; in 10 h there are (60 s/min)(60
min/h)(10 h) = 36,000 s—so enter the value “3.6e4”.
5. Next, at the bottom of this window click on the button labeled “Add curve”.
6. On the right portion of the screen will appear a concentration profile for this particular diffusion
situation. A diamond-shaped cursor will appear at the upper left-hand corner of the resulting curve. Click and drag
this cursor down the curve to the point at which the number below “Concentration:” reads “0.25 wt%”. Then read
the value under the “Distance:”. For this problem, this value (the solution to the problem) is 0.91 mm.
Factors That Influence Diffusion
5.20 &LWHWKHYDOXHVRIWKHGLIIXVLRQFRHIILFLHQWVIRUWKHLQWHUGLIIXVLRQRIFDUERQLQERWKͣ-iron (BCC) and
ͥ-iron (FCC) at 900°C. Which is larger? Explain why this is the case.
Solution
We are asked to compute the diffusion coefficients of C in both D and J iron at 900qC. Using the data in
Table 5.2,
The D for diffusion of C in BCC D iron is larger, the reason being that the atomic packing factor is smaller
than for FCC J iron (0.68 versus 0.74—Section 3.4); this means that there is slightly more interstitial void space in
the BCC Fe, and, therefore, the motion of the interstitial carbon atoms occurs more easily.
5.23 At what temperature will the diffusion coefficient for the diffusion of zinc in copper have a value of
–16
2.6 × 10 m2/s? Use the diffusion data in Table 5.2.
Solution
We are asked to calculate the temperature at which the diffusion coefficient for the diffusion of Zn in Cu
has a value of 2.6 u 1016 m2/s. Solving for T from Equation 5.9a
and using the data from Table 5.2 for the diffusion of Zn in Cu (i.e., D0 = 2.4 u 105 m2/s and Qd = 189,000 J/mol) ,
we get
= 901 K = 628qC
Note: This problem may also be solved using the “Diffusion” module in the VMSE software. Open the “Diffusion”
module, click on the “D vs 1/T Plot” submodule, and then do the following:
1. In the left-hand window that appears, there is a preset set of data for the diffusion of Zn in Cu system.
Therefore, click on the "Cu-Ni" box.
2. In the column on the right-hand side of this window the activation energy-preexponential data for this
problem. In the window under “D0” the preexponential value, “2.4e-5”, is displayed. Next just below the “Qd” the
activation energy value (in KJ/mol), “189” is displayed. In the "Temperature Range" window minimum ("T Min")
and maximum ("T Max") temperatures are displayed, in both qC and K. The default values for minimum and
maximum temperatures are 800ºC and 1140ºC, respectively.
3. Next, at the bottom of this window, click the "Add Curve" button.
4. A log D versus 1/T plot then appears, with a line for the temperature dependence of the diffusion
coefficient for Zn in Cu. At the top of this curve is a diamond-shaped cursor. We want to click-and-drag this cursor
down the line (to the right) to the point at which the entry under the “Diff Coeff (D):” label reads 2.6 u 10-16 m2/s.
As we drag the cursor to the right, the value of the diffusion coefficient continues to decrease, but only to about 1.5
u 10-14 m2/s. This means that the temperature at which the diffusion coefficient is 2.6 u 10-16 m2/s is lower than
800ºC. Therefore, let us change the T Min value to 600ºC. After this change is made click again on the "Add
Curve", and a new curve appears. Now click and drag the cursor to the right until "2.6 u 10-16 m2/s " is displayed
under "Diff Coeff (D)". At this time under the "Temperature (T)" label is displayed the temperature—in this case
"902 K".
5.32 Carbon is allowed to diffuse through a steel plate 10 mm thick. The concentrations of carbon at the
two faces are 0.85 and 0.40 kg C/cm3 Fe, which are maintained constant. If the preexponential and activation
energy are 5.0 × 10–7 m2/s and 77,000 J/mol, respectively, compute the temperature at which the diffusion flux is 6.3
× 10–10 kg/m2.s.
Solution
This problem asks that we compute the temperature at which the diffusion flux is 6.3 u 1010 kg/m2-s.
Combining Equations 5.2 and 5.8 yields
Taking natural logarithms of both sides of this equation yields the following expression:
Or
And solving for T from this expression leads to
Now, incorporating values of the parameters in this equation provided in the problem statement leads to the
following:
= 884 K = 611qC
1084°C (1357 K). Assume an energy for vacancy formation of 0.90 eV/atom.
(b) Repeat this calculation at room temperature (298 K).
(c) What is ratio of Nv /N(1357 K) and Nv /N(298 K)?
Solution
(a) In order to compute the fraction of atom sites that are vacant in copper at 1357 K, we must employ
Equation 4.1. As stated in the problem, Qv = 0.90 eV/atom. Thus,
(b) We repeat this computation at room temperature (298 K), as follows:
(c) And, finally the ratio of Nv /N(1357 K) and Nv /N(298 K) is equal to the following:
4.13 What is the composition, in atom percent, of an alloy that consists of 92.5 wt% Ag and 7.5 wt% Cu?
Solution
In order to compute composition, in atom percent, of a 92.5 wt% Ag-7.5 wt% Cu alloy, we employ
Equation 4.6 given the atomic weights of silver and copper (found on the inside of the book's cover):
AAg = 107.87 g/mol
ACu = 63.55 g/mol
These compositions in atom percent are determined as follows:
= 87.9 at%
= 12.1 at%
4.17 What is the composition, in atom percent, of an alloy that contains 33 g of copper and 47 g of zinc?
Solution
The concentration of an element in an alloy, in atom percent, may be computed using Equation 4.5a.
However, it first becomes necessary to compute the number of moles of both Cu and Zn, using Equation 4.4.
Atomic weights of copper and zinc (found on the inside of the book's cover) are as follows:
ACu = 63.55 g/mol
AZn = 65.41 g/mol
Thus, the number of moles of Cu is just
Likewise, for Zn
Now, use of Equation 4.5a yields
Also,
4.20 Calculate the number of atoms per cubic meter in Pb.
Solution
This problem calls for a determination of the number of atoms per cubic meter for lead. In order to solve
this problem, one must employ Equation 4.2,
The density of Pb (from the table inside of the front cover) is 11.35 g/cm3, while its atomic weight is 207.2 g/mol.
Thus,
4.21 Calculate the number of atoms per cubic meter in Cr.
Solution
This problem calls for a determination of the number of atoms per cubic meter for chromium. In order to
solve this problem, one must employ Equation 4.2,
The density of Cr (from the table inside of the front cover) is 7.19 g/cm3, while its atomic weight is 52.00 g/mol.
Thus,
4.25 Calculate the unit cell edge length for an 80 wt% Ag20 wt% Pd alloy. All of the palladium is in
solid solution, the crystal structure for this alloy is FCC, and the room-temperature density of Pd is 12.02 g/cm3.
Solution
In order to solve this problem it is necessary to employ Equation 3.8; in this expression density and atomic
weight will be averages for the alloy—that is
Inasmuch as the unit cell is cubic, then VC = a3, then
And solving this equation for the unit cell edge length, leads to
Expressions for Aave and Uaveare found in Equations 4.11a and 4.10a, respectively, which, when
incorporated into the above expression yields
Since the crystal structure is FCC, the value of n in the above expression is 4 atoms per unit cell. The
atomic weights for Ag and Pd are 107.87 and 106.4 g/mol, respectively (Figure 2.8), whereas the densities for the
Ag and Pd are 10.49 g/cm3 (inside front cover) and 12.02 g/cm3. Substitution of these, as well as the concentration
values stipulated in the problem statement, into the above equation gives
4.26 Some hypothetical alloy is composed of 25 wt% of metal A and 75 wt% of metal B. If the densities of
metals A and B are 6.17 and 8.00 g/cm3, respectively, and their respective atomic weights are 171.3 and 162.0
g/mol, determine whether the crystal structure for this alloy is simple cubic, face-centered cubic, or body-centered
cubic. Assume a unit cell edge length of 0.332 nm.
Solution
In order to solve this problem it is necessary to employ Equation 3.8; in this expression density and atomic
weight will be averages for the alloy—that is
Inasmuch as for each of the possible crystal structures, the unit cell is cubic, then VC = a3, or
Now, in order to determine the crystal structure it is necessary to solve for n, the number of atoms per unit
cell. For n =1, the crystal structure is simple cubic, whereas for n values of 2 and 4, the crystal structure will be
either BCC or FCC, respectively. When we solve the above expression for n the result is as follows:
Expressions for Aave and Uave are found in Equations 4.11a and 4.10a, respectively, which, when incorporated into
the above expression yields
Substitution of the concentration values (i.e., CA = 25 wt% and CB = 75 wt%) as well as values for the other
parameters given in the problem statement, into the above equation gives
= 1.00 atom/unit cell
Therefore, on the basis of this value, the crystal structure is simple cubic.
4.37 Iron and vanadium both have the BCC crystal structure, and V forms a substitutional solid solution
for concentrations up to approximately 20 wt% V at room temperature. Compute the unit cell edge length for a 90
wt% Fe–10 wt% V alloy.
Solution
This problems asks that we compute the unit cell edge length for a 90 wt% Fe-10 wt% V alloy. First of all,
the atomic radii for Fe and V (using the table inside the front cover) are 0.124 and 0.132 nm, respectively. Also,
using Equation 3.8 it is possible to compute the unit cell volume, and inasmuch as the unit cell is cubic, the unit cell
edge length is just the cube root of the volume. However, it is first necessary to calculate the density and average
atomic weight of this alloy using Equations 4.10a and 4.11a. Inasmuch as the densities of iron and vanadium are
7.87g/cm3 and 6.10 g/cm3, respectively, (as taken from inside the front cover), the average density is just
= 7.65 g/cm3
And for the average atomic weight
= 55.32 g/mol
Now, VC is determined from Equation 3.8 as (taking the number of atoms per unit cell, n = 2, since the crystal
structure of the alloy is BCC)
= 2.40 u 1023 cm3/unit cell
And, finally, because the unit cell is of cubic symmetry
= 2.89 u 108 cm = 0.289 nm
4.42 (a) For a given material, would you expect the surface energy to be greater than, the same as, or less
than the grain boundary energy? Why?
(b) The grain boundary energy of a small-angle grain boundary is less than for a high-angle one. Why is
this so?
Answer
(a) The surface energy will be greater than the grain boundary energy. For grain boundaries, some atoms
on one side of a boundary will bond to atoms on the other side; such is not the case for surface atoms. Therefore,
there will be fewer unsatisfied bonds along a grain boundary.
(b) The small-angle grain boundary energy is lower than for a high-angle one because more atoms bond
across the boundary for the small-angle, and, thus, there are fewer unsatisfied bonds.
Diffusion Mechanisms
5.3 (a) Compare interstitial and vacancy atomic mechanisms for diffusion.
(b) Cite two reasons why interstitial diffusion is normally more rapid than vacancy diffusion.
Answer
(a) With vacancy diffusion, atomic motion is from one lattice site to an adjacent vacancy. Self-diffusion
and the diffusion of substitutional impurities proceed via this mechanism. On the other hand, atomic motion is from
interstitial site to adjacent interstitial site for the interstitial diffusion mechanism.
(b) Interstitial diffusion is normally more rapid than vacancy diffusion because: (1) interstitial atoms,
being smaller, are more mobile; and (2) the probability of an empty adjacent interstitial site is greater than for a
vacancy adjacent to a host (or substitutional impurity) atom.
5.9 A sheet of steel 2.5-mm thick has nitrogen atmospheres on both sides at 900°C and is permitted to
achieve a steady-state diffusion condition. The diffusion coefficient for nitrogen in steel at this temperature is 1.85
× 10–10 m2/s, and the diffusion flux is found to be 1.0 × 10–7 kg/m2 . s. Also, it is known that the concentration of
nitrogen in the steel at the high-pressure surface is 2 kg/m3. How far into the sheet from this high-pressure side will
the concentration be 0.5 kg/m3? Assume a linear concentration profile.
Solution
This problem is solved by using Equation 5.2 in the form
If we take CA to be the point at which the concentration of nitrogen is 2 kg/m3, then it becomes necessary to solve
the above equation for xB, as
Assume xA is zero at the surface, in which case
5.14 An FCC iron–carbon alloy initially containing 0.55 wt% C is exposed to an oxygen-rich and virtually
carbon-free atmosphere at 1325 K (1052°C). Under these circumstances the carbon diffuses from the alloy and
reacts at the surface with the oxygen in the atmosphere—that is, the carbon concentration at the surface position is
maintained essentially at 0 wt% C. (This process of carbon depletion is termed decarburization.) At what position
will the carbon concentration be 0.25 wt% after a 10-h treatment? The value of D at 1325 K is 3.3 × 10–11 m2/s.
Solution
This problem asks that we determine the position at which the carbon concentration is 0.25 wt% after a 10-
h heat treatment at 1325 K when C0 = 0.55 wt% C. From Equation 5.5
Thus,
Using data in Table 5.1 and linear interpolation
z erf (z)
0.40 0.4284
z 0.4545
0.45 0.4755
which leads to,
z = 0.4277
This means that
And, finally
= 9.32 u 104 m = 0.932 mm
Note: This problem may also be solved using the “Diffusion” module in the VMSE software. Open the “Diffusion”
module, click on the “Diffusion Design” submodule, and then do the following:
1. Enter the given data in left-hand window that appears. In the window below the label “D Value” enter
the value of the diffusion coefficient—viz. “3.3e-11”.
2. In the window just below the label “Initial, C0” enter the initial concentration—viz. “0.55”.
3. In the window the lies below “Surface, Cs” enter the surface concentration—viz. “0”.
4. Then in the “Diffusion Time t” window enter the time in seconds; in 10 h there are (60 s/min)(60
min/h)(10 h) = 36,000 s—so enter the value “3.6e4”.
5. Next, at the bottom of this window click on the button labeled “Add curve”.
6. On the right portion of the screen will appear a concentration profile for this particular diffusion
situation. A diamond-shaped cursor will appear at the upper left-hand corner of the resulting curve. Click and drag
this cursor down the curve to the point at which the number below “Concentration:” reads “0.25 wt%”. Then read
the value under the “Distance:”. For this problem, this value (the solution to the problem) is 0.91 mm.
Factors That Influence Diffusion
5.20 &LWHWKHYDOXHVRIWKHGLIIXVLRQFRHIILFLHQWVIRUWKHLQWHUGLIIXVLRQRIFDUERQLQERWKͣ-iron (BCC) and
ͥ-iron (FCC) at 900°C. Which is larger? Explain why this is the case.
Solution
We are asked to compute the diffusion coefficients of C in both D and J iron at 900qC. Using the data in
Table 5.2,
The D for diffusion of C in BCC D iron is larger, the reason being that the atomic packing factor is smaller
than for FCC J iron (0.68 versus 0.74—Section 3.4); this means that there is slightly more interstitial void space in
the BCC Fe, and, therefore, the motion of the interstitial carbon atoms occurs more easily.
5.23 At what temperature will the diffusion coefficient for the diffusion of zinc in copper have a value of
–16
2.6 × 10 m2/s? Use the diffusion data in Table 5.2.
Solution
We are asked to calculate the temperature at which the diffusion coefficient for the diffusion of Zn in Cu
has a value of 2.6 u 1016 m2/s. Solving for T from Equation 5.9a
and using the data from Table 5.2 for the diffusion of Zn in Cu (i.e., D0 = 2.4 u 105 m2/s and Qd = 189,000 J/mol) ,
we get
= 901 K = 628qC
Note: This problem may also be solved using the “Diffusion” module in the VMSE software. Open the “Diffusion”
module, click on the “D vs 1/T Plot” submodule, and then do the following:
1. In the left-hand window that appears, there is a preset set of data for the diffusion of Zn in Cu system.
Therefore, click on the "Cu-Ni" box.
2. In the column on the right-hand side of this window the activation energy-preexponential data for this
problem. In the window under “D0” the preexponential value, “2.4e-5”, is displayed. Next just below the “Qd” the
activation energy value (in KJ/mol), “189” is displayed. In the "Temperature Range" window minimum ("T Min")
and maximum ("T Max") temperatures are displayed, in both qC and K. The default values for minimum and
maximum temperatures are 800ºC and 1140ºC, respectively.
3. Next, at the bottom of this window, click the "Add Curve" button.
4. A log D versus 1/T plot then appears, with a line for the temperature dependence of the diffusion
coefficient for Zn in Cu. At the top of this curve is a diamond-shaped cursor. We want to click-and-drag this cursor
down the line (to the right) to the point at which the entry under the “Diff Coeff (D):” label reads 2.6 u 10-16 m2/s.
As we drag the cursor to the right, the value of the diffusion coefficient continues to decrease, but only to about 1.5
u 10-14 m2/s. This means that the temperature at which the diffusion coefficient is 2.6 u 10-16 m2/s is lower than
800ºC. Therefore, let us change the T Min value to 600ºC. After this change is made click again on the "Add
Curve", and a new curve appears. Now click and drag the cursor to the right until "2.6 u 10-16 m2/s " is displayed
under "Diff Coeff (D)". At this time under the "Temperature (T)" label is displayed the temperature—in this case
"902 K".
5.32 Carbon is allowed to diffuse through a steel plate 10 mm thick. The concentrations of carbon at the
two faces are 0.85 and 0.40 kg C/cm3 Fe, which are maintained constant. If the preexponential and activation
energy are 5.0 × 10–7 m2/s and 77,000 J/mol, respectively, compute the temperature at which the diffusion flux is 6.3
× 10–10 kg/m2.s.
Solution
This problem asks that we compute the temperature at which the diffusion flux is 6.3 u 1010 kg/m2-s.
Combining Equations 5.2 and 5.8 yields
Taking natural logarithms of both sides of this equation yields the following expression:
Or
And solving for T from this expression leads to
Now, incorporating values of the parameters in this equation provided in the problem statement leads to the
following:
= 884 K = 611qC